Received: November 2018
DOI 10.17677/fn20714807.2019.01.01
Fluorine Notes, 2019, 122, 1-2
Stereocontrolled synthesis of N-heterocyclic fluorine-containing β-amino acid derivatives
Lamiaa Ouchakour,1,3 Melinda Nonn,1,2,3 Loránd Kiss1,3*
1Institute of Pharmaceutical Chemistry, University of Szeged, H-6720 Szeged, Eötvös u. 6, Hungary
2MTA-SZTE Stereochemistry Research Group, Hungarian Academy of Sciences, H-6720 Szeged, Eötvös u. 6, Hungary
3University of Szeged, Interdisciplinary Excellence Centre, Institute of Pharmaceutical Chemistry
E-mail: kiss.lorand@pharm.u-szeged.hu; kiss.lorand00@gmail.com;
web: http://www2.pharm.u-szeged.hu/gyki
Graphical abstract:
Abstract:
A stereocontrolled procedure is reported for the access of various fluorine-containing piperidine and azepane β-amino esters. The synthetic protocol starts from readily available unsaturated cycloalkene β-amino acids and is based on oxidative cleavage of the ring olefin bond followed by ring closing of the diformyl intermediates in the presence of some fluorine-containing amines across reductive amination.
Keywords: fluorine, stereocontrol, reductive amination, ring expansion, azaheterocycle, amino acid
Introduction
Cyclic β-amino acids are considered to be novel compounds in the field of synthetic and medicinal chemistry and they have exerted increasing interest during the past twenty years because of the importance of some antifungal, antibacterial or analgetic small molecules. For example, (1R,2S)-2-aminocyclopentanecarboxylic acid (cispentacin, 1), (1R,2S)-2-amino-4-methylenecyclopentanecarboxylic acid (icofungipen, 2), BAY Y9379 (3), (2R,3S)-3-aminooxetane-2-carboxylic acid (oxetin, 4) or tilidin (5) are a few of some highly functionalized derivatives in drug research (Figure 1, 1-5). These compounds are of high significance because they are key elements of various bioactive products with antitumoral, antibacterial, antiviral and cardioprotective activities. As conformationally restricted building blocks, these small molecules are of appreciable importance for the synthesis of peptides and, accordingly, they exert a considerable impact in the fields of biomolecules and drug design.1
Figure 1. Some small cyclic β-amino acids with pharmaceutical relevance.
Azaheterocyclic β-amino acids, which express high biological relevance, represent an important class of compounds in both medicinal and organic chemistry. Thus, several piperidine and pyrrolidine β-amino acid derivatives exhibit antiviral or antibacterial activities (Figure 2, 6-8).1
Figure 2. Some azaheterocyclic β-amino acids with biological importance.
Organofluorine compounds have generated increasing attention in the field of pharmaceuticals and agrochemicals over the last decade.2 Among the ever-increasing number of fluorine-containing biomolecules or building blocks, a number of fluorinated open-chain α-, β- or γ-amino acids exhibit antitumoral or antibiotic properties.3
Figure 3. Several molecules with fluoroamine or trifluoroamine units.
Molecules containing β-fluorinated or β-trifluorinated amine moieties are important entities in pharmaceutical chemistry or agrochemistry.2,4 Accordingly, fluorine-containing five- or six-membered azaheterocycles, which are components in drugs such as MK-0657, MK-0731 or neceprevir, are of high importance in medicinal chemistry.5 However, fluorine-containing seven-membered N-heterocycles are relatively less abundant in the literature.
Despite of this, they might gain high relevance in the future, which is due to the important role of some functionalized counterparts in drug research.6 Fluorine-containing tetrahydroisoquinoline derivatives and other types of N-fluoroalkylated molecular scaffolds are known to possess important biologically properties.7 β-Fluoroamine or -trifluoroamine units are also present in versatile fluorine-containing amino acid derivatives of biological relevance (Figure 3, 9-13).8
Fluorine-containing saturated N-heterocycles are of special significance, since introduction of one or more fluorine atoms into the skeleton of an azaheterocycle can increase lipophilicity and metabolic stability. Moreover, fluorine substitution may reduce basicity and, accordingly, it provides better bioavailability to a certain molecule. Moreover, perfluorocarbons are characterized by biological inertness, however they exhibit intense hydrophobic and lipophilic effects.
Results and Discussion
Because of the high biological importance of saturated azaheterocycles, our current ai was to combine β-amino acid and organofluorine molecular entities and synthesize novel molecular structures. This manuscript is intended to offer an insight into the extension of our earlier work9a based on stereocontrolled synthesis of fluorine-containing piperidine or azepane β-amino acid derivatives. The synthetic approach included the use of some commercially available fluorinated or polyfluorinated primary amines and was based on the oxidative ring cleavage of unsaturated cyclic β-amino esters through the ring olefin bond (through vicinal diol). It is followed by ring closing by double reductive amination giving the products across ring expansion of the diformyl intermediates.9
Cyclopentene cis-β-amino acid (±)-14 was first converted by esterification according to the route described earlier10, followed by N-protection and cis-dihydroxylation with the OsO4/NMO system to the corresponding dihydroxylated cis amino ester (±)-15. This diol was submitted to oxidative ring opening with NaIO4 in THF/H2O affording the corresponding acylic diformyl amino ester (±)-16. This relatively unstable dialdehyde derivative was further used in the forthcoming step without isolation. Thus (±)-16 was subjected to double-reductive amination with various commercially available fluorinated primary amines such as 2,2,3,3,4,4,5,5,5-nonafluoropentan-1-amine, 2,2,3,3,4,4,5,5,6,6,7,7,7-tridecafluoroheptan-1-amine and 1,1,1-trifluoropropan-2-amine. The reaction was induced with NaBH3CN in the presence of CH2Cl2. Ring closing involves reductive amination and gives the corresponding fluorinated or perfluorinated cis β-amino esters with a piperidine ring system ((±)-17a-c) (Scheme 1).
Scheme 1. Synthesis of fluorinated piperidine cis-β-amino esters.
amino ester |
fluorine-containing amine |
product |
yield (two steps) |
![]() |
![]() |
![]() |
13%, (±)-17a |
![]() |
![]() |
![]() |
14%, (±)-17b |
![]() |
![]() |
![]() |
21%, (±)-17c |
Table 1. Synthesis of piperidine cis-β-amino esters 17a-c.
Since the configuration of the stereocenters at C-1 and C-2 of amino esters (±)-14 and (±)-15 were not affected during the ring closing procedure, the integrity of the configuration of the chiral centers in (±)-17a-c was conserved, that is configurations are predetermined by the structure of the starting materials.
Accordingly, cis amino ester provided the corresponding piperidine derivative with the carboxylate and carbamate functions at C-3 and C-4 in a cis relative arrangement (Scheme 1, Table 1). Noteworthy, that the cyclization reaction performed with 1,1,1-trifluoropropan-2-amine as the amine source containing a chiral center yielded only a single piperidine compound ((±)-17b).
In continuation, cis isomer (±)-15 was converted to cyclopentene trans-β-amino acid (±)-189a,10 in an analogous way. Namely, compound (±)-15 with the ester and the carbamate groups in a trans relationship was subjected to oxidative ring opening with NaIO4.
Scheme 2. Synthesis of fluorinated piperidine trans-β-amino esters.
amino ester |
fluorine-containing amine |
product |
yield (two steps) |
![]() |
![]() |
![]() |
15%, (±)-20a |
![]() |
![]() |
![]() |
26%, (±)-20b |
Table 2. Synthesis of piperidine trans-β-amino esters 20a-b.
The formed instable dialdehyde intermediate (±)-19 was treated with fluorinated amines and NaBH3CN in CH2Cl2 without isolation furnishing fluorinated piperdine trans amino esters (±)-20a,b (Scheme 2, Table 2). Note, contrary to its cis counterpart compound (±)-20a proved to be unstable. Again, the reaction took place with stereocontrol with the conservation of the configuration of the chiral centers C-1 and C-2 of (±)-18 corresponding to C-3 and C-4 in products (±)-20a,b (Scheme 2, Table 2).
The synthetic procedure presented above was further extended to the efficient access of fluorinated azepane β-aminocarboxylic esters. For example, cis-β-aminocyclohex-4-enecarboxylates (±)-21 undergoing esterification, N-benzoylation and cis-dihydroxylation with NMO/OsO49a,11 resulted in the corresponding vicinal cis diol derivatives (±)-22. Subsequently, this dihydroxylated ester was transformed via oxidative ring cleavage and ring expansion. The latter step involved reductive amination with commercially available fluorinated or perfluorinated primary amines (see Table 3) in the presence of NaBH3CN. The process proceeds through stereocontrol affording the corresponding cis azepane amino esters (±)-24a-e (Scheme 3).
Thus, applying the oxidative ring opening/ring closure with reductive amination protocol, a series of mono-, di- or trifluorinated as well as perfluorinated seven-membered N-heterocyclic cis amino esters could be accessed.
Scheme 3. Synthesis of fluorinated azepane β-amino esters.
Conclusions
A simple stereocontrolled synthetic route has been developed for the preparation of novel fluorine-containing six- and seven-membered N-heterocyclic β-amino esters, based on olefin bond oxidative ring cleavage of cyclopentene and cyclohexene β-amino acids, followed by ring closing with double reductive amination of diformyl intermediates in the presence of commercially available primary fluoroamines. Since the stereocenters of the starting carbocyclic β-amino esters are not affected during the process, they will predetermine the configuration of the chiral centers in the azaheterocyclic products.
amino ester |
fluorine-containing amine |
product |
yield (two steps) |
![]() |
![]() |
![]() |
15%, (±)-24a |
![]() |
![]() |
![]() |
12%, (±)-24b |
![]() |
![]() |
![]() |
27%, (±)-24c |
![]() |
![]() |
![]() |
38%, (±)-24d |
![]() |
![]() |
![]() |
27%, (±)-24e |
Table 3. Synthesis of azepane β-amino esters 24a-e.
Experimental Section
General procedure for the synthesis of fluorine-containing N-heterocyclic β-amino esters by oxidative ring cleavage followed by reductive amination
To a stirred solution of β-amino ester (2 mmol) NaIO4 (1.5 equiv) was added in THF/H2O (25 mL/2 mL). After stirring for 1 h at 20 °C under argon atmosphere, H2O was added (40 mL). Then the mixture was extracted with CH2Cl2 (3×20 mL) and the combined extract was dried over Na2SO4. The crude dialdehyde product was immediately used for reductive amination without purification. Fluorinated or polyfluorinated amines (1 equiv) were added to the solution in CH2Cl2 (30 mL) and the mixture was stirred at 20 °C for 10 minutes. Next NaBH3CN (1 equiv) and AcOH (2 drops) were added and stirring was continued for another 4 h at 20 °C. Then the reaction mixture was diluted with H2O (20 mL) and extracted with CH2Cl2 (3×20 mL). The combined organic layer was dried (Na2SO4) and concentrated under vacuum. The crude product was purified by column chromatography on silica gel (n-hexane/EtOAc).
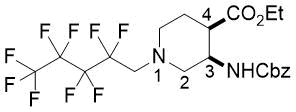
(3R*,4R*)-Ethyl 3-(((benzyloxy)carbonyl)amino)-1-(2,2,3,3,4,4,5,5,5-nonafluoropentyl)piperidine-4-carboxylate, (±)-17a
Yellow oil; yield: 13% (126 mg); Rf = 0.75 (n-hexane/acetone 4:1); 1H-NMR (500 MHz, CDCl3): δ = 1.22 (t, J = 7.0 Hz, 3H, CH3), 1.73-1.80 (m, 1H, H-5), 1.93-2.05 (m, 1H, H-5), 2.45-2.55 (m, 2H, H-2, H-6), 2.67-2.72 (m, 1H, H-4), 2.88-2.94 (m, 2H, H-2, H-6), 3.06 (t, J = 16.1 Hz, 2H, NCH2), 4.05-4.15 (m, 2H, CH2CH3) 4.28-4.34 (m, 1H, H-3), 5.07 (s, 2H, OCH2), 5.56 (brs, 1H, N-H), 7.28-7.39 (m, 5H, Ar-H); 13C-NMR (126 MHz, CDCl3): δ = 14.1, 23.8, 43.6, 47.8, 52.9, 56.7 (t, 2JC,F = 22.5 Hz, C(CF2)5), 58.8, 60.9, 66.7, 107.6-120.8 (m, 4 C, (CF2)5CF3), 127.9, 128.0, 128.5, 136.6, 155.6, 172.4; 19F-NMR (471 MHz, CDCl3): δ = -80.9 (t, J = 9.4 Hz), -115.8, -124.1, -126.2. MS: (ESI) m/z = 539.73 (M+1).
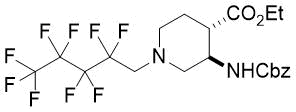
(3R*,4S*)-Ethyl 3-(((benzyloxy)carbonyl)amino)-1-(2,2,3,3,4,4,5,5,5-nonafluoropentyl)piperidine-4-carboxylate, (±)-20a
Yellow oil; yield: 15% (210 mg); Rf = 0.41 (n-hexane/EtOAc 4:1); 1H-NMR (400 MHz, CDCl3): δ = 1.23 (t, J = 7.0 Hz, 3H, CH3), 1.70-2.04 (m, 2H, H-5), 2.41-3.17 (m, 6H, H-2, H-6, NCH2), 4.07-4.21 (m, 3H, CH2CH3, H-4), 5.10 (s, 2H, OCH2), 5.21-5.34 (m, 1H, H-3), 7.28-7.47 (m, 6H, Ar-H, N-H); 19F-NMR (376 MHz, CDCl3): δ = -80.9 (t, J = 7.5 Hz), -115.8, -124.1, -126.1.
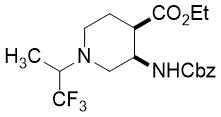
(3R*,4R*)-Ethyl 3-(((benzyloxy)carbonyl)amino)-1-(1,1,1-trifluoropropan-2-yl)piperidine-4-carboxylate, (±)-17b
Yellow oil; yield: 14% (111 mg); Rf = 0.24 (n-hexane/EtOAc 4:1); 1H-NMR (400 MHz, CDCl3): δ = 1.08-1.19 (m, 6H, CH3), 1.53-1.62 (m, 1H, H-5), 1.82-1.98 (m, 1H, H-5), 2.53-2.95 (m, 4H, H-2, H-6), 3.35-3.51 (m, 1H, H-4), 3.88-4.03 (m, 2H, CH2CH3), 4.03-4.27 (m, 2H, H-3, NCH), 4.95-5.08 (m, 2H, OCH2), 6.79 (brs, 1H, N-H), 7.29-7.41 (m, 5H, Ar-H), 13C-NMR (100 MHz, CDCl3): δ = 14.7, 14.8, 24.2, 44.2, 48.6, 49.7, 52.9, 59.5, 60.0 (q, 2JC,F = 23.3 Hz, CCF3), 60.6, 66.0, 117.7, 128.4, 128.6, 129.1, 138.2, 151.9, 172.8; 19F-NMR (376 MHz, CDCl3): δ = -70.1 (t, J = 11.3 Hz); MS: (ESI) m/z = 403.41 (M+1).
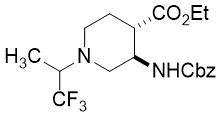
(3R*,4S*)-Ethyl 3-(((benzyloxy)carbonyl)amino)-1-(1,1,1-trifluoropropan-2-yl)piperidine-4-carboxylate, (±)-20b
Colorless oil; yield: 26% (300 mg); Rf = 0.48 (n-hexane/EtOAc 1:4); 1H-NMR (500 MHz, CDCl3): δ = 1.17-1.27 (m, 6H, CH3), 1.83-1.96 (m, 2H, H-5), 2.35-2.88 (m, 4H, H-2, H-6), 2.99-3.02 (m, 2H, H-4, NCH), 3.97-4.08 (m, 1H, H-3), 4.10-4.20 (m, 2H, CH2CH3), 5.09 (m, 2H, OCH2), 5.16 (brs, 1H, N-H), 7.28-7.40 (m, 5H, Ar-H); 13C-NMR (126 MHz, CDCl3): δ = 10.5, 14.2, 26.2, 45.3, 46.3, 48.9, 52.2, 60.5 (q, 2JC,F = 26.9 Hz, CCF3), 60.8, 66.8, 125.7 (q, 1JC,F =288.5 Hz, CF3) , 128.2, 128.5, 136.4, 155.4, 172.7; 19F-NMR (471 MHz, CDCl3): δ = -71.4.
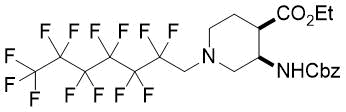
(3R*,4R*)-Ethyl 3-(((benzyloxy)carbonyl)amino)-1-(2,2,3,3,4,4,5,5,6,6,7,7,7-tridecafluoroheptyl)piperidine-4-carboxylate, (±)-17c
Yellow oil; yield: 21% (125 mg); Rf = 0.58 (n-hexane/EtOAc 2:1); 1H-NMR (500 MHz, CDCl3): δ = 1.22 (t, J = 7.0 Hz, 3H, CH3), 1.74-1.83 (m, 1H, H-5), 1.93-2.04 (m, 1H, H-5), 2.45-2.56 (m, 2H, H-2, H-6), 2.67-2.74 (m, 1H, H-4), 2.88-2.97 (m, 2H, H-2, H-6), 3.07 (t, J = 15.5 Hz, 2H, N-CH2), 4.06-4.14 (m, 2H, CH2CH3) 4.29-4.37 (m, 1H, H-3), 5.07 (s, 2H, OCH2), 5.57 (brs, 1H, N-H), 7.27-7.39 (m, 5H, Ar-H); 13C-NMR (126 MHz, CDCl3): δ = 14.1, 23.8, 43.6, 47.8, 52.9, 56.7 (t, 2JC,F = 22.0 Hz, C(CF2)5), 58.0, 60.9, 66.7, 108.1-119.5 (m, 6 C, (CF2)5CF3), 127.9, 128.1, 128.5, 136.5, 155.7, 172.4; 19F-NMR (471 MHz, CDCl3): δ = -80.8 (t, J = 9.4 Hz), -115.4, -122.0, -122.8, -123.0, -126.1; MS: (ESI) m/z = 639.27 (M+1).
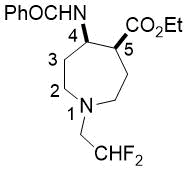
Ethyl (4S*, 5S*)-5-benzamido-1-(2,2-difluoroethyl)azepane-4-carboxylate, (±)-24a
Colorless oil; yield: 15% (90 mg); Rf =0.48 (n-hexane/EtOAc 2:1); 1H-NMR (500 MHz, CDCl3): δ = 1.24-1.28 (m, 5H, CH3, H-6), 1.82-1.94 (m, 2H, H-3), 2.08-2.29 (m, 2H, H-2), 2.73-2.83 (m, 3H, H-7, H-4), 2.84-2.94 (m, 3H, CH2CHF2, H-5), 4.12-4.23 (m, 2H, CH2), 4.65-4.76 (brs. 1H, NH), 5.60-6.25 (tt, 1H, CHF2, 1J = 56.17 Hz, 2J = 54.76 Hz) 7.39-7.84 (m, 5H, Ar-H); 13C-NMR (126 MHz, CDCl3): δ = 14.1, 27.9, 29.7, 31.9, 47.1, 48.2 (t, 2JC,F = 25.3 Hz, CCHF2), 50.0, 52.3, 52.8, 60.4, 60.6, 60.7, 60.8, 115.4 (t, 1JC,F = 241.2 Hz, CHF2), 126.9, 127.2, 128.5, 128.6, 131.4, 134.5, 166.4, 173.4; 19F-NMR (471 MHz, CDCl3): δ = -123.9 Hz; MS: (ESI) m/z = 355.88 (M+1).
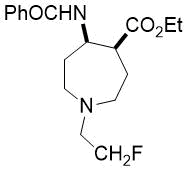
Ethyl (4S*,5S*)-5-benzamido-1-(2-fluoroethyl)azepane-4-carboxylate, (±)-24b
Colorless oil; yield: 12% (80 mg); Rf =0.5 (n-hexane/EtOAc 2:1); 1H-NMR (500 MHz, CDCl3): δ = 1.23-1.25 (m, 3H, CH3), 1.26-1.28 (m, 2H, H-3), 1.84-1.93 (m, 2H, H-6), 2.13-2.27 (m, 2H, H-2), 2.65-2.75 (m, 2H, H-7), 2.79-2.93 (m, 3H, H-4, CH2-CH2F), 4.08-4.19 (m, 2H, CH2CH3), 4.41-4.57 (dt, 2H, CH2F, 1J = 48.49 Hz, 2J = 27.01 Hz), 4.78-4.86 (m, 1H, H-5), 7.38-7.50 (m, 3H, Ar-H), 7.63-7.70 (brs. 1H, NH), 7.74-7.82 (m, 5H, Ar-H); 13C-NMR (126 MHz, CDCl3): δ = 14.1, 28.1, 29.7, 31.5, 47.9, 49.6, 51.7, 51.9, 58.5 (d, 2JC,F = 19.3 Hz, CCH2F), 60.7, 81.9 (d 1JC,F = 168.9 Hz, CH2F), 126.9, 127.6, 128.4, 128.7, 131.3, 134.6, 166.3, 173.4; 19F-NMR (471 MHz, CDCl3): δ = -216.3 Hz; MS: (ESI) m/z = 337.57 (M+1).
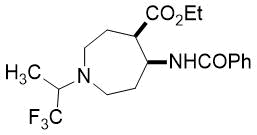
Ethyl (4R*, 5S*)-5-benzamido-1-(1,1,1-trifluoropropan-2-yl)azepane-4-carboxylate, (±)-24c
Colorless oil, yield: 27% (100mg); Rf = 0.51 (n-hexane/EtOAc, 6:1); 1H-NMR (500 MHz, CDCl3): δ = 1.17-1.22 (d, 3H, CH3), 1.28 (t, J = 7.08 Hz, 3H, CH3), 1.81-1.90 (m, 2H, H-6, H-3), 1.98-2.09 (m, 1H, H-6), 2.26-2.37 (m, 1H, H-3), 2.65-2.91 (m, 4H, H-2, H-7), 2.91-3.00 (m, 1H, H-4), 3.12-3.25 (m, 1H, H-5), 4.11-4.24 (m, 2H, CH2), 4.46-4.58 (m, 1H, NCH), 7.38-7.45 (t, 3H, Ar-H); 7.46-7.51 (brs. 1H, NH), 7.70-7.81 (d, 2H, Ar-H); 13C-NMR (126 MHz, CDCl3): δ = 10.0, 14.1, 27.9, 32.7, 45.9, 47.2, 48.5, 50.9, 60.5, 60.8 (q, 2JC,F = 27.0 Hz, CCF3), 126.9, 127.8 (1JC,F = 285 Hz, CF3),, 128.5, 131.3, 134.6, 166.2, 173.2; 19F-NMR (471 MHz, CDCl3): δ = -72.7 Hz; MS: (ESI) m/z = 387.62 (M+1).
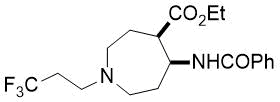
(4R*, 5S*)-Ethyl 5-benzamido-1-(3,3,3-trifluoropropyl)azepane-4-carboxylate, (±)-24d
Colorless oil, yield: 38% (240mg); Rf = 0.35 (n-hexane/EtOAc, 6:1); 1H-NMR (500MHz, CDCl3): δ = 1.26 (t, J = 7.0 Hz, 3H, CH3), 1.82-1.93 (m, 2H, H-6, H-3), 2.03-2.16 (m, 1H, H-6), 2.17-2.30 (m, 3H, H-3, CH2CF3), 2.51-2.61 (m, 2H, H-2), 2.66-2.79 (m, 4H, H-7, NCH2), 2.79-2.88 (m, 1H, H-4), 4.09-4.22 (m, 2H, OCH2), 4.62-4.74 (m, 1H, H-5), 7.40-7.43 (brs. 1H, NH), 7.43-7.77 (m, 5H, Ar-H); 13C-NMR (126 MHz, CDCl3): δ = 14.1, 27.7, 31.9, 32. (q, 2JC,F = 26.8 Hz, CCF3), 47.1, 50.1, 51.0, 51.3 (d, 3JC,F = 2.7 Hz, CCCF3), 51.6, 60.6, 126.4 (q, 1JC,F =277.0 Hz, CF3), 126.9, 128.5, 131.4, 134.6, 166.4, 173.4; 19F-NMR (471 MHz, CDCl3): δ = -64.9 Hz; MS: (ESI) m/z = 387.61 (M+1).
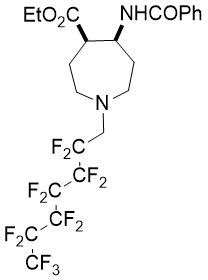
(4R*,5S*)-Ethyl 5-benzamido-1-(2,2,3,3,4,4,5,5,6,6,7,7,7-tridecafluorohepty)azepane-4-carboxylate, (±)-24e
Colorless oil; yield: 27% (200 mg); Rf = 0.28 (n-hexane/EtOAc 2:1); 1H-NMR (500 MHz, CDCl3): δ = 1.26 (t, J = 7.16 Hz, 3H, CH3), 1.84-1.93 (m, 2H, H-3, H-6), 2.10-2.34 (m, 2H, H-3, H-6), 2.77-2.87 (m, 2H, H-2), 2.87-2.92 (m, 1H, H-4), 2.92-2.98 (m, 2H, H-7), 3.09-3.29 (m, 2H, CH2CF2), 4.12-4.20 (m, 2H, CH2), 4.61-4.74 (m, 1H, H-5), 7.33-7.44 (m, 3H, Ar-H), 7.45-7.54 (brs. 1H, NH), 7.74-7.81 (m, 2H, Ar-H); 13C-NMR (126 MHz, CDCl3): δ = 14.1, 27.9, 32.0, 46.9, 50.1, 52.7, 53.4, 57.6 (t, 2JC,F = 20.5 Hz, CCHF2), 60.6, 111.0-121.2 (m, 6C, (CF2)5CF3), 126.9, 128.4, 131.3, 134.5, 166.47, 173.1; 19F-NMR (471 MHz, DMSO-d6): δ = -81.1 (t, J = 9.94 Hz), -117.3, -122.0, -122.8, -123.5, -126.1; MS: (ESI) m/z = 624 (M+1).
Acknowledgments
We are grateful to the Hungarian Research Foundation (NKFIH Nos. K 115731, K 129049 and K 119282) for financial support. The financial support of the GINOP-2.3.2-15-2016-00034 project is also acknowledged. This research was supported by the EU-funded Hungarian grant EFOP-3.6.1-16-2016-00008. Ministry of Human Capacities, Hungary grant 20391-3/2018/FEKUSTRAT is also acknowledged.
References:
- (a) Kiss, L.; Mándity, I. M.;· Fülöp, F. Amino Acids 2017, 49, 1441; (b) Kiss, L.; Fülöp, F. Chem. Rev. 2014, 114, 1116. (c) Grygorenko, O. O. Tetrahedron 2015, 71, 5169. (d) Risseeuw, M.; Overhand, M.; Fleet, G. W. J.; Simone, M. I. Amino Acids 2013, 45, 613.
- (a) Zhou, Y.; Wang, Y.; Gu, Z.; Wang, S.; Zhu, W.; Acena, J. L.; Soloshonok, V. A.; Izawa, K.; Liu, H. Chem. Rev. 2016, 116, 442; (b) Wang, J.; Sánchez-Roselló, M.; Aceña, J. L.; del Pozo, C.; Sorochinsky, A. E.; Fustero, S.; Soloshonok, V. A.; Liu, H. Chem. Rev. 2014, 114, 2432. (c) Fluorine in Pharmaceutical and Medicinal Chemistry: From Biophysical Aspects to Clinical Applications, Imperial College Press, London 2012. Edited by Gouverneur, V. and Müller, K. (d) Purser, S.; Moore, P. R.; Swallow, S.; Gouverneur, V. Chem. Soc. Rev. 2008, 37, 320.
- (a) Ouchakour, L.; Ábrahámi, R. A.; Forró, E.; Haukka, M.; Fülöp, F.; Kiss, L. Eur. J. Org. Chem. 2018, doi: 10.1002/ejoc.201801540; (b) Mikami, K.; Fustero, S.; Sanchez-Rosello, M.; Acena, J. L.; Soloshonok, V. A.; Sorochinsky, A. Synthesis 2011, 304; (c) Vogensen, S. B.; Jørgensen, L.; Madsen, K. K.; Jurik, A.; Borkar, N.; Rosatelli, E.; Nielsen, B.; Ecker, G. F.; Schousboe, A.; Clausen, R. P. Bioorg. Med. Chem. 2015, 23, 2480; (d) Acena, J. L.; Sorochinsky, A. Soloshonok, V. A.; Synthesis 2012, 1591, (e) Salwiczek, M.; Nyakatura, E. K.; Gerling, U. I. M.; Ye, S.; Koksch, B. Chem. Soc. Rev. 2012, 41, 2135; (f) Qiu, X. L.; Qing, F. L. Eur. J. Org. Chem. 2011, 3261.
- (a) Hu, X. G.; Hunter, L. Beilstein J. Org. Chem. 2013, 9, 2696; (b) Chen, P.; Liu, G. Eur. J. Org. Chem. 2015, 4295; (c) Jeanmart, S.; Edmunds, A. J. F.; Lamberth, C.; Pouliot, M. Bioorg. Med. Chem. 2016, 24, 317; (d) Kalow, J. A.; Schmitt, D. E.; Doyle, A. G. J. Org. Chem. 2012, 77, 4177.
- (a) Dolfen, J.; Kenis, S.; van Hecke, K.; De Kimpe, N.; D’hooghe, M. Chem. Eur J. 2014, 20, 10650; (b) van Hende, E.; Verniest, G.; Thuring, J. W.; Macdonald, G.; Deroose, F.; De Kimpe, N. Synlett 2009, 1765; (c) Piron, K.; Verniest, G.; van Hende, E.; De Kimpe, N. Arkivoc 2012, v, 6; (d) Orliac, A.; Routier, J.; Charvillon, F. B.; Sauer, W. H. B.; Bombrun, A.; Kulkarni, S. S.; Pardo, D. G.; Cossy, J. Chem. Eur. J. 2014, 20, 3813; (e) Fustero, S.; Sanz-Cervera, J. F.; Aceña, J. L.; Sánchez-Roselló, M. S. Synlett 2009, 525; (f) Verniest, G.; Piron, K.; Van Hende, E.; Thuring, J. W.; Macdonald, G.; Deroose, F.; De Kimpe, N. Org. Biomol. Chem. 2010, 8, 2509; (g) Wu, L.; Chen, P.; Liu, G. Org. Lett. 2016, 18, 960; (h) Yan, N.; Fang, Z.; Liu, Q. Q.; Guo, X. H.; Hu, X. G. Org. Biomol. Chem. 2016, 14, 3469; (i) Artamonov, O. S.; Slobodyanyuk, E. Y.; Volochnyuk, D. M.; Komarov, I. V.; Tolmachev, A. A.; Mykhailiuk, P. K. Eur. J. Org. Chem. 2014, 3592.
- (a) Beng, T. K.; Wilkerson-Hill, S. M.; Sarpong, R. Org. Lett. 2014, 16, 916; (b) Patel, A. R.; Liu, F. Tetrahedron Lett. 2013, 69, 744.
- (a) Ábrahámi, R. A.; Fustero, S.; Fülöp, F.; Kiss, L. Synlett 2018, 29, 2066; (b) Schmitt, S.; Colloc'h, N.; Perrio, C. Eur. J. Med. Chem. 2015, 90, 742; (c) Toyama, H.; Sato, S.; Shirakawa, H.; Komai, M.; Hashimoto, Y.; Fujii, S. Bioorg. Med. Chem. Lett. 2016, 26, 1817; (d) Riss, P. J.; Hummerich, R.; Schloss, P. Org. Biomol. Chem. 2009, 7, 2688.
- (a) Cho, J.; Nishizono, N.; Iwahashi, N.; Saigo, K.; Ishida, Y. Tetrahedron2013, 69, 9252; (b) Gu, X. H.; Zong, R.; Kula, N. S.; Baldessarini, R. J.; Neumeyera, J. L. Bioorg. Med. Chem. Lett. 2001, 11, 3049; (c) Yang, X.; Chen, Z.; Cai, Y.; Huang, Y. Y.; Shibata, N. Green Chem. 2014, 16, 4530; (d) Carboni, A.; Dagousset, G.; Magnier, E.; Masson, G. Org. Lett.2014, 16, 1240.
- (a) Ábrahámi, R. A.; Kiss, L.; Barrio, P.; Fülöp, F. Tetrahedron 2016, 72, 7526; (b) Ábrahámi, R. A., Kiss, L.; Fustero, S.; Fülöp, F. Synthesis 2017, 49, 1206; (c) Kiss, L.; Forró, E.; Fülöp, F. Beilstein J. Org. Chem. 2015, 11, 596; (d) Kazi, B.; Kiss, L.; Forró, E.; Fülöp, F. Tetrahedron Lett. 2010, 51, 82; (e) Kiss, L.; Kazi, B.; Forró, E.; Fülöp, F. Tetrahedron Lett. 2008, 49, 339.
- (a) Cherepanova, M.; Kiss, L.; Forró, E.; Fülöp, F. Eur. J. Org. Chem. 2014, 403; (b) Benedek, G.; Palkó, M.; Wéber, E.; Martinek, T. A.; Forró, E.; Fülöp, F. Eur. J. Org. Chem. 2008, 3724; (c) Remete, A. M.; Nonn, M.; Fustero, S.; Haukka, M.; Fülöp, F.; Kiss, L. Beilstein J. Org. Chem. 2017, 13, 2364; (d) Nonn, M.; Kiss, L.; Forró, E.; Mucsi, Z.; Fülöp, F. Tetrahedron 2011, 67, 4079.
- (a) Cherepanova, M.; Kiss, L.; Fülöp, F. Tetrahedron 2014, 70, 2515; (b) Kiss, L.; Forró, E.; Martinek, T. A.; Bernáth, G.; De Kimpe, N.; Fülöp, F. Tetrahedron 2008, 64, 5036.
Supporting Information with NMR spectra in PDF version.
Recommended for publication by Prof. József Rábai.
Fluorine Notes, 2019, 122, 1-2